Projects
All research projects in the KT Consortium are conducted in close collaboration with the industrial company members of the consortium.
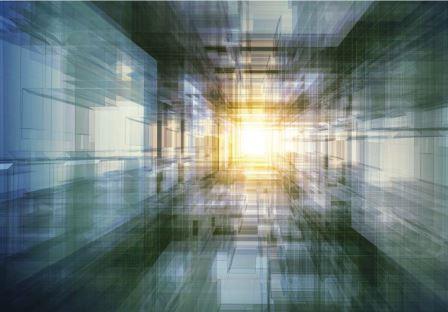
Current projects
Objective
In close synergy and collaboration with CERE we are working on a number of projects, aiming at a further development and understanding of advanced thermodynamic models like CPA and PC-SAFT for various applications (see under description of the project).
Many of these projects are of "exploratory" character. Upon completion and/or/if/when results are in form suitable for practical engineering applications, they may be included in ICAS, if appropriate.
Background
This is a generic project (actually a list of diverse projects under the same headline), in close collaboration and synergy with CERE.
The project
Applications we consider for CPA and PC-SAFT:
- mixtures with hydrogen bonding compounds like water and alcohols/glycols
http://www.cere.dtu.dk/Research-and-Projects/Research-Projects/CHIGP - electrolytes
http://www.cere.dtu.dk/Research-and-Projects/PhD-Projects/Archive--PhD/Development-of-an-Electrolyte-CPA-Equation-of-state-for-App-in-the-Petroleum-and-Chemical-Industries
http://www.cere.dtu.dk/research-and-projects/phd-projects/development-of-the-electrolyte-cpa-equation-of-state - asphaltenes
http://www.cere.dtu.dk/research-and-projects/phd-projects/modelling-of-asphaltene-systems-with-association-models - surface and interfacial tensions
http://www.cere.dtu.dk/research-and-projects/phd-projects/phase-behavior-of-inhomogeneous-fluids - chemicals of relevance to CO2 capture
http://www.cere.dtu.dk/research-and-projects/phd-projects/thermodynamic-modeling-of-ionic-liquid-containing-co2-gas-hydrate-formation-systems - simulation of biofuel processes
http://www.cere.dtu.dk/research-and-projects/phd-projects/thermodynamics-design-simulation-and-benchmarking-of-biofuel-processes - critical point investigations
http://www.cere.dtu.dk/research-and-projects/phd-projects/application-of-the-cubic-plus-association-equation-of-state-in-reservoir-simulation
Contact.
Georgios Kontogeorgis Professor Department of Chemical and Biochemical Engineering Phone: +45 45252859 gk@kt.dtu.dk
Contact
Xiaodong Liang Associate Professor Department of Chemical and Biochemical Engineering Phone: +45 45252877 xlia@kt.dtu.dk
Objective
The project’s overall target is to arrive at a fundamental understanding of electrolyte thermodynamics and thus enable the engineering of a new generation of useful, physically sound models for electrolyte solutions.
These models should be general and applicable to a very wide range of conditions so that they can be potentially used for a wide range of applications.
Electrolyte solutions are present almost anywhere and find numerous applications in physical sciences including chemistry, geology, material science, medicine, biochemistry and physiology as well as in many engineering fields especially chemical & biochemical, electrical and petroleum engineering.
In all these applications the thermodynamics plays a crucial role over wide ranges of temperature, pressure and composition. As the subject is important, a relatively large body of knowledge has been accumulated with lots of data and models.
However, disappointingly the state-of-the art thermodynamic models used today in engineering practice are semi-empirical and require numerous experimental data. They lack generality and have not enhanced our understanding of electrolyte thermodynamics.
Going beyond the current state of the art, we will create the scientific foundation for studying, at their extremes, both “primitive” and “non-primitive” approaches for electrolyte solutions and identify strengths and limitations.
The ambition is to make new advances to clarify major questions and misunderstandings in electrolyte thermodynamics, some remaining for over 100 years, which currently prevent real progress from being made, and create a new paradigm which will ultimately pave the way for the development of new engineering models for electrolyte solutions.
This is a risky, ambitious and crucial task, but a successful completion will have significant benefits in many industrial sectors as well as in environmental studies and biotechnology.
The project is founded on a well-structured connection between fundamental understanding and macroscopic modeling.
At a practical level, the ambition is to yield a roadmap of what is possible and what not and when for engineering applications in electrolyte solutions and also, at a more fundamental level, to make new advances which will clarify some of the major confusions and misunderstandings in electrolyte thermodynamics which currently prevent real progress from taking place.
External collaborators:
Professor Athanassios Z. Panagiotopoulos (University of Princeton, USA)
Professor Ioannis Economou (NCSR “Demokritos”, Greece)
Professor Jean-Charles de Hemptinne (IFP Energies Nouvelles, France)
Contact.
Georgios Kontogeorgis Professor Department of Chemical and Biochemical Engineering Phone: +45 45252859 gk@kt.dtu.dk
Contact
Xiaodong Liang Associate Professor Department of Chemical and Biochemical Engineering Phone: +45 45252877 xlia@kt.dtu.dk
Contact
Nicolas von Solms Professor Department of Chemical and Biochemical Engineering nvs@kt.dtu.dk
Contact
Kaj Thomsen Department of Chemical and Biochemical Engineering kth@kt.dtu.dk
Ionic liquid (IL) database and property models update IL-based tools development (collaborate with Guoliang) IL-based aqueous biphasic system (ABS) study and design
Properties of ILs are required for the design of products and processes involving ILs.
Although innumerable ILs may be generated through the combination of a variety of cations, anions and substituents, only a small part of them have been reported to exist (have been synthesized).
The available experimental data are generally limited and sometimes even contradictory. A detailed knowledge about the properties of ILs is critically important, especially for ILs not yet available.
Based on collected experimental data from numerous literature sources, a series of group contribution (GC)-based models have been developed for estimating various properties.
On the other hand, prediction of thermodynamic behavior is essential for the early design stage of separation processes including solvent selection, process optimization and its performance evaluation. A UINIFAC-IL-Gas model has been developed for such a purpose.
The developed database and property models can be used easily in IL-based tools. In addition, IL-based ABS has many advantages in bio-separations, but its optimal design is very difficult due to the limited property knowledge.
A systematic study of these systems is of great importance for the applications of ABS involving ILs.
Main supervisor:
Georgios M. Kontogeorgis
Co- supervisor:
Xiaodong Liang
Contact.
Georgios Kontogeorgis Professor Department of Chemical and Biochemical Engineering Phone: +45 45252859 gk@kt.dtu.dk
Contact
Xiaodong Liang Associate Professor Department of Chemical and Biochemical Engineering Phone: +45 45252877 xlia@kt.dtu.dk
The Fermentation Based Biomanufacturing initiative offers a comprehensive research-based education and training program in Fermentation Based Biomanufacturing (FBM) at the Technical University of Denmark (DTU) with the mission to deliver world-class research-based education in biomanufacturing, tailor-made towards the needs of the fermentation industry.
The project aims to improve our understanding of enzyme stability and broaden the field of potential applications for biocatalysis.
Accelerated Innovation in Manufacturing Biologics (AIM-Bio) project funded by the Novo Nordisk Fundation brings together two leading academic institutions, NC State University (US) and the Technical University of Denmark, aiming to create an international collaborative enterprise engaged in education, lifelong learning, and process research and development in biomanufacturing science and technology.